Stanford Medicine Advances Molecular Biology with TF Activation Profiling Plate Array
- Signosis
- Apr 14, 2023
- 4 min read
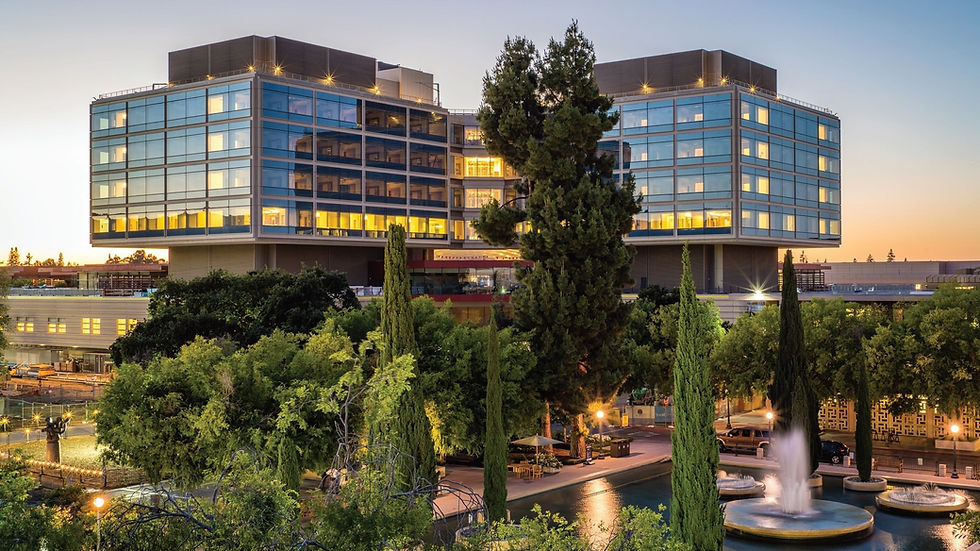
Stanford Medicine has made significant strides in the field of molecular biology with the use of the TF Activation Profiling Plate Array with Nuclear Extract. This technology allows for the analysis of transcription factor (TF) activation in a high-throughput manner, providing insights into the regulation of gene expression.
In particular, Stanford Medicine has customized the hybridization plate and probes for 8 TFs, enabling the profiling of TF activation in a specific biological context. Additionally, the team has also developed customized TF Activation Profiling Plate Arrays for 6 TFs, further expanding the range of potential applications.
One area where this technology has been successfully applied is in the study of pancreatic cancer suppression. By leveraging a hyperactive p53 variant, the team has elucidated how p53 acts at the cellular level to suppress the development of pancreatic ductal adenocarcinomas. The team found that p53 limits ADM accumulation, suppresses PanIN cell proliferation, and suppresses KRAS signaling in PanINs, thus providing key new understanding of p53 function in PDAC.
Another sector in which Stanford Medicine has made significant progress is in the field of RNA structure and function prediction. By utilizing high-throughput biochemical techniques and modeling frameworks, the team has been able to map the relationship between RNA sequence, structure, and function. These techniques have the potential to revolutionize our understanding of RNA biology and enable the development of more accurate models for predicting the structure and function of RNA.
Finally, the team has also made important contributions to our understanding of heart development and congenital heart disease. By generating single-cell chromatin accessibility maps of human fetal heart tissues and interpreting deep learning models of cell-type-resolved chromatin accessibility profiles, the team has identified disruption of cell type-specific regulatory elements in CHD. These findings have important implications for the diagnosis and treatment of heart disease.
Overall, Stanford Medicine's use of the TF Activation Profiling Plate Array with Nuclear Extract has enabled important advances in the fields of cancer biology, RNA biology, and developmental biology, providing insights into the regulation of gene expression and the mechanisms underlying disease.

The vast majority of human pancreatic ductal adenocarcinomas (PDACs) harbor TP53 mutations, underscoring p53’s critical role in PDAC suppression. PDAC can arise when pancreatic acinar cells undergo acinar-to-ductal metaplasia (ADM), giving rise to premalignant pancreatic intraepithelial neoplasias (PanINs), which finally progress to PDAC. The occurrence of TP53 mutations in late-stage PanINs has led to the idea that p53 acts to suppress malignant transformation of PanINs to PDAC. However, the cellular basis for p53 action during PDAC development has not been explored in detail. Here, we leverage a hyperactive p53 variant—p5353,54—which we previously showed is a more robust PDAC suppressor than wild-type p53, to elucidate how p53 acts at the cellular level to dampen PDAC development. Using both inflammation-induced and KRASG12D-driven PDAC models, we find that p5353,54 both limits ADM accumulation and suppresses PanIN cell proliferation and does so more effectively than wild-type p53. Moreover, p5353,54 suppresses KRAS signaling in PanINs and limits effects on the extracellular matrix (ECM) remodeling. While p5353,54 has highlighted these functions, we find that pancreata in wild-type p53 mice similarly show less ADM, as well as reduced PanIN cell proliferation, KRAS signaling, and ECM remodeling relative to Trp53-null mice. We find further that p53 enhances chromatin accessibility at sites controlled by acinar cell identity transcription factors. These findings reveal that p53 acts at multiple stages to suppress PDAC, both by limiting metaplastic transformation of acini and by dampening KRAS signaling in PanINs, thus providing key new understanding of p53 function in PDAC.
RNAs are central to fundamental biological processes in all known organisms. The set of possible intramolecular interactions of RNA nucleotides defines the range of alternative structural conformations of a specific RNA that can coexist, and these structures enable functional catalytic properties of RNAs and/or their productive intermolecular interactions with other RNAs or proteins. However, the immense combinatorial space of potential RNA sequences has precluded predictive mapping between RNA sequence and molecular structure and function. Recent advances in high-throughput approaches in vitro have enabled quantitative thermodynamic and kinetic measurements of RNA–RNA and RNA–protein interactions, across hundreds of thousands of sequence variations. In this Review, we explore these techniques, how they can be used to understand RNA function and how they might form the foundations of an accurate model to predict the structure and function of an RNA directly from its nucleotide sequence. The experimental techniques and modelling frameworks discussed here are also highly relevant for the sampling of sequence–structure–function space of DNAs and proteins.
To define the multi-cellular epigenomic and transcriptional landscape of cardiac cellular development, we generated single-cell chromatin accessibility maps of human fetal heart tissues. We identified eight major differentiation trajectories involving primary cardiac cell types, each associated with dynamic transcription factor (TF) activity signatures. We contrasted regulatory landscapes of iPSC-derived cardiac cell types and their in vivo counterparts, which enabled optimization of in vitro differentiation of epicardial cells. Further, we interpreted sequence based deep learning models of cell-type-resolved chromatin accessibility profiles to decipher underlying TF motif lexicons. De novo mutations predicted to affect chromatin accessibility in arterial endothelium were enriched in congenital heart disease (CHD) cases vs. controls. In vitro studies in iPSCs validated the functional impact of identified variation on the predicted developmental cell types. This work thus defines the cell-type-resolved cis-regulatory sequence determinants of heart development and identifies disruption of cell type-specific regulatory elements in CHD.
Comentarios